Near-field measurements 101
Today’s electromagnetic simulation and analysis tools have become quite sophisticated and capable of modeling complex devices in real world scenarios, so the question often arises, Why do I need to test my antenna? Simulations still depend on assumptions regarding the environment in which the device will be used, which may or may not be accurate. And then there are the unexpected “unknowns” that we did not take into consideration during the design process. Measurements are used to verify the design of our device in the environment in which it is intended to operate.
As antennas are typically designed to operate at great distances apart, this is ultimately how we would like to test our device, in what is deemed the radiated “far-field”. However, this can often become impractical due to other limiting factors such as available space and extraneous interfering signals if testing outdoors. Another approach is to measure the antenna radiated energy very close to the device in the radiating “near-field” and then use a mathematical algorithm to transform the measured data to the radiated far-field. The space required to make a near-field measurement is much less, allowing even electrically large antennas to be tested in an indoor test facility.
Radiating antenna Fields
The reactive near-field is located very close to the radiating antenna surface, typically within a distance a of wavelength or two. Within this regions, the fields are changing very quickly due to evanescent waves created by surface currents, and do not propagate. At a distance of a couple wavelengths, we enter the radiating near-field region. Here the EM fields are well behaved but are still represented by spherical waves, and the antenna beam has not yet been fully formed. The nearfield region extends to a distance of 2D2/l, at which point we can say the fields now resemble plane waves. This region is referred to at the far-field (or Fraunhoffer) region and is where the antenna beam has now been developed.
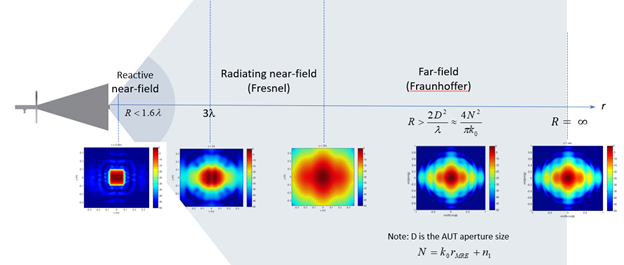
Antenna far-field characteristics must be measured at a sufficiently large distance between the source antenna and the AUT. This distance must be greater than the larger of the two inner limits of the far zones of the transmitting and receiving antennas, i.e., the two antennas must be in each other’s far zones.
How Far is far-field?
In practice, antenna EM fields radiating in 3-D space can be represented by spherical wave fronts when the observation point is sufficiently far from the source. At large distances from the source antenna, the curvature of the phase front is small at the aperture of the Antenna Under Test (AUT) and approximates a uniform plane wave. If the distance from the source is equal or greater than the inner boundary of the far-field region of the source, Rmin > 2D2max/l, then the maximum phase difference between the actual incident field and its far-zone approximation does not exceed 22.5 degrees (i.e.
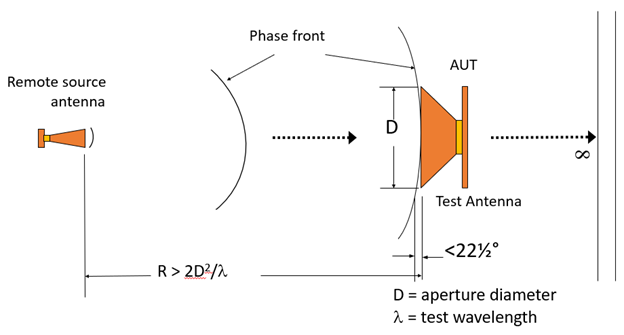
The above requirement leads to a major difficulty in antenna measurements – large separation distances are required between the source antenna and the AUT. The larger the electrical size of the AUT, the larger the measurement site.
Near-field measurement Concept
Near-field measurement systems offer an alternative solution for characterizing antennas at reduced distances. In this case, the distance between the source (or probe) antenna and AUT is only several wavelengths.
The near-field measurement concept requires collecting the energy in the radiated near-field of the antenna and then using a mathematical algorithm to spatially transform the results to the far-field. Because this algorithm requires complex data (i.e. I/Q), measurements must include both amplitude and phase information. In addition, a significant amount of the radiated energy needs to be collected to provide accurate results, which requires 2D measurements. While the data processing takes only a few minutes to perform, the near-field measurement time may be significant. However, the end result provides 2D far-field pattern information in a much more time efficient manner than making the equivalent measurement using a far-field system.
Near-field Scan Geometries
Near-field measurement systems are offered in several different configurations and are classified by the geometry of the measured near-field surface. Traditional near-field geometries include planar, cylindrical, and spherical. Other more complex geometries, such as conical or non-conformal, are possible but are less common.
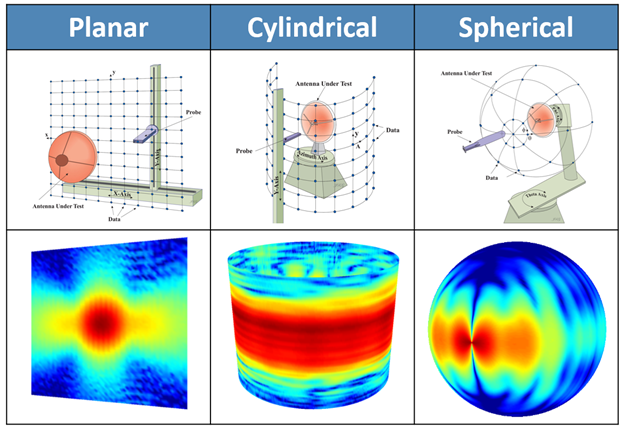
Every near-field measurement system provides at least two measurement axes: a primary and secondary axis, commonly referred to as the scan and step axis. Nearfield systems can also be classified as single or multi-probe systems. In a single probe system, motion axes are used for both the primary and secondary axes. In a multi-probe system, the primary axes is replaced with a multi-probe array, which performs an electronic scan while a motion axis is still used for the secondary axis. However, we will limit this near-field discussion to single probe systems as many of the basic principles also apply to multi-probe systems.
In a single probe planar near-field (PNF) configuration, the AUT remains stationary and a robotic scanner is used to move a probe antenna in a XY plane located in front of the AUT. As the plane is limited to collecting energy only in front of the antenna, this type of system has a limited Field of View (FOV) and is best suited for characterizing highly directive antennas (i.e. gain > 15 dBi) over far-field angles of less than +/- 80 degrees.
A cylindrical near-field (CNF) system can provide 360 degrees of far-field angle coverage in one coordinate plane, but still has limited coverage in the other plane. This type of system is best suited for characterizing fan beam antennas or when more than +/- 80 degrees of angle coverage is required in a single coordinate plane. This measurement is implemented by adding a rotation axis for the AUT and using a single linear axis to move the probe in the vertical direction.
A spherical near-field (SNF) system is used to characterize an antenna over wide angle ranges and is required when measuring a low gain antenna. A single probe spherical near-field system requires two motion axes to rotate the AUT while the probe remains stationary. While a spherical near-field measurement system can collect all of the radiating energy from the AUT over a complete 360-degree sphere, the SNF-FF transform requires measurements of both probe polarization orientations where the other geometry solutions do not.
Near-field Measurement Considerations
There are some additional considerations that need to be taken into account when making near-field measurements.
First off, the mathematical transform requires measuring all the “significant” AUT radiated energy. Not collecting enough energy can result in truncation errors, which is an artifact of the transform process where aliased energy is directed back into the far-field antenna pattern at boresight. This can have a direct impact on gain measurement accuracy. Rule of thumb for determining if enough of the significant energy has been collected is to examine the measured near-field amplitude data and verify the data at the surface boundaries it is at least -30 dB from the near-field peak, and ≤ -50 dB to minimize the truncation error.
Another consideration is the data sampling interval over the near-field measurement surface. Nyquist sampling theory requires that signals must be sampled at twice the signal frequency to reconstruct an image free signal. This equates to a sampling interval of l/2 or less to guarantee an image free far-field region of +/-90 degrees. However, the sampling interval may be increased to speed up measurement time if collecting data for a reduced far-field angle extent, as is often the case when characterizing satellite antennas.
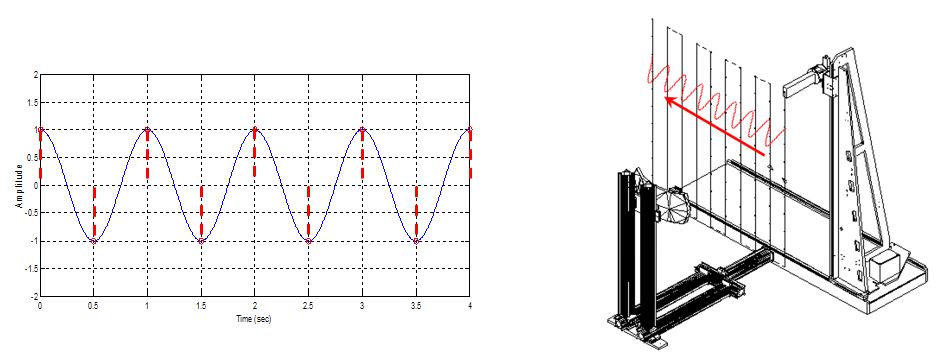
nother requirement for making near-field measurement is probe positioning accuracy, which should be greater than l/50 and at least l/100 for accurate sidelobe measurements. For higher frequency measurements, this requirement can exceed the manufacturing mechanical tolerances of the positioning system! To combat this issue, many near-field system providers (including NPM) offer solutions for improving positioning accuracy. The NPM solution provides real-time positioning correction to facilitate measurements at millimeter frequencies and higher.
Since a near-field measurement involves two antennas (the AUT and the near-field probe), the resulting measurement is a convolution of both antenna responses. To obtain the response of just the AUT, the probe contribution must be removed from the acquired data. This can be accomplished provided the probe pattern is known and is one of the reasons why open-ended waveguides are often used as near-field probes. In addition to having a broad and well-behaved co-pol response, the pattern can easily be calculated based on the probe waveguide dimensions.
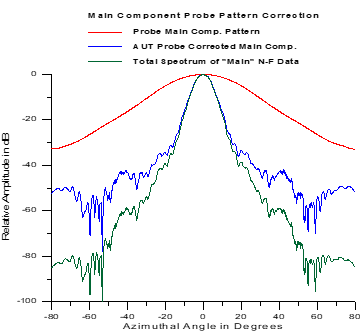
When making near-field measurements, both probe polarization senses are not necessarily required to provide far-field results. However, there are a few exceptions. Measurements of both polarizations ARE required for (1) acquiring the far-field cross pol pattern, (2) accurate sidelobe information in the intercardinal region, (3) measuring a circularly polarized antenna using a linear polarization nearfield probe, and (4) when making spherical nearfield measurements.
Near-field measurements offer an alternative solution to far-field measurements for characterizing antennas and have many advantages. However, this is not a “one-size-fits-all” and there are applications where far-field measurements may provide a better solution. NPM can work with you to help determine the correct solution for your measurement application and requirements.